Volume 4 - Year 2017 - Pages 19 - 22
DOI: 10.11159/jbeb.2017.002
Pattern Change of Flow Diverters due to Bending
Lilla Asztalos1, Péter Nagy2, Eszter Bognár2,3, István Szikora2
1 Department of Materials Science and Engineering, Faculty of Mechanical Engineering
Budapest University of Technology and Economics
Bertalan Lajos str. 7. H-1111, Budapest, Hungary
Asztalos.lilla92@gmail.com
2 Department of Neurointerventions, National Institute of Clinical Neurosciences
Amerikai str. 57. H-1145 Budapest, Hungary
Npeter@eik.bme.hu; H13424szi@ella.hu
3 MTA–BME Research Group for Composite Science and Technology
Muegyetem rkp. 3., H-1111 Budapest, Hungary
Eszter@eik.bme.hu
Abstract - Flow diverters have a very short history, their research and clinical use for intracranial aneurysms spread in the last 10 years. Because of the novelty of these devices, there are many fields to research, that correlate with the effect of flow diverters. Such research may improve the safety and efficacy of the technique. These devices are very flexible and can be slightly bent, but the surface mesh pattern changes frequently, so the metallic surface area surrounding the aneurysm is not constant. Current manufacturers are working with several musters, however, neither an ideal surface structure nor a standard index-number for the optimal MSA (Metallic Surface Area) have been determined. The aim of this study is to test flow diverter devices from two different manufacturers at fixed bending angles at various points obtain the mesh pattern, and calculate the area coverage. Based on the results, an objective measurement for the local values can be defined. The ideal measurement for coverage can be decided by analysing the microscopic images of the stents at the marked points and at the determined angles.
Keywords: Flow diverter, metallic surface area (MSA), intracranial aneurysm
© Copyright 2017 Authors This is an Open Access article published under the Creative Commons Attribution License terms. Unrestricted use, distribution, and reproduction in any medium are permitted, provided the original work is properly cited.
Date Received: 2015-09-21
Date Accepted: 2016-11-31
Date Published: 2017-09-05
1. Introduction
Flow diversion has been recently introduced into the endovascular treatment of intracranial aneurysms. Long-term clinical tests prove that flow diverters (FD) can be safely and effectively used with a lower complication rate comparable to other methods. [1,2] FD-s are flexible, self-expanding, tubular devices that provide a high density wire mesh across the orifice of the aneurysm when implanted within the parent artery. [3] The hydraulic resistance created by the FD will reduce the dynamic fluid exchange between the parent artery and aneurysm sac promoting aneurysm thrombosis. After successful aneurysm thrombosis, the construct becomes progressively incorporated into the parent artery, providing a homogeneous layer of tissue separating the aneurysm cavity from the parent artery’s lumen. [4] The primary effect of the FD-s is related to the hydraulic resistance that is a function of the MSA, the percentage of surface covered by the metallic wires of the FD across the orifice presented in Eq.1 as:

FDs are made of nickel-titanium or cobalt-chromium alloys. In addition, platinum is used to provide radiopacity. The construct is packaged collapsed on a delivery wire and contained within a delivery sheath. When the construct is fully expanded, it foreshortens approximately 2.5 times to its nominal length. [5] Most manufacturers guarantee 30-55% MSA with full expansion to their nominal diameter compared to 6-10% produced by non-flow diverting stents. FDs are available in different diameters from 2.5-5 mm having a nominal length of 10-50 mm. The pore size (the average area of the tetragon of the wire mesh) at nominal diameter is 0.02-0.05 mm2. [6]
Shapiro et al. examined the variable porosity of an FD by placing it in pipe sections of different diameters. The results confirmed that the compression of FD had a larger impact on the pattern, thereby to effectiveness factor: the MSA. [7]
Due to specific characteristics of intracranial vascular anatomy, instead of straight vessels, most aneurysms are located on curved sections of arteries. Subsequently, FD-s are bended to variable degrees once implanted. As bending of the FD may also impact its wire mesh pattern, it is important to analyse the resulting MSA at different bending angles at different points along the circumference of the device.
2. Material and Methods
2. 1. The flow diverters
During this research 3 FDs were tested. Details of the samples are listed in Table 1. Stereomicroscopic images (Olympus SZX16, Olympus, Tokyo, Japan, magnification: 20×, Figure 1-3) demonstrate the wire pattern when fully expanded and not bended.
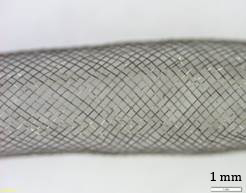
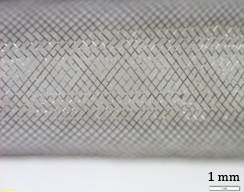
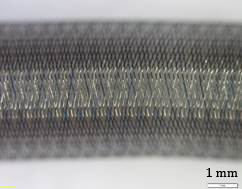
2. 2. Methodology
To observe the change of the mesh pattern caused by bending, a holder was created to hold the sample at different angles without affecting the wire pattern. Both ends of the sample was placed in a silicone tube as the silicone surface gives good adhesion, which aids attachment of the sample at that position during bending. The angulation was stabilized by wires bent to the required angles. The angled section of the FD remained free from both wires and silicone tubes for unrestricted observation. Considering the nominal diameter of the samples, a 5 mm internal diameter silicone tube was chosen, making sure that compression did not changed the wire pattern during the investigation. Then, the samples were put in the tube and formed a bent position as required for measurement. Figure 4 shows an illustration of the holder.
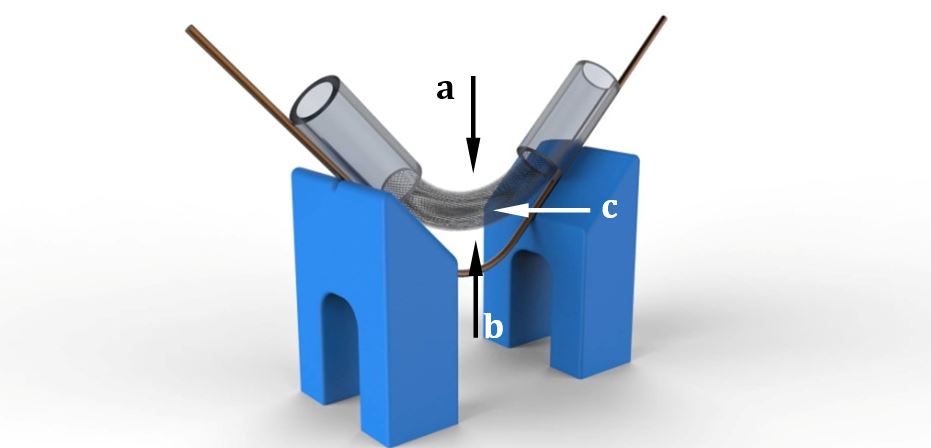
Images of the samples were taken using a stereo microscope at all chosen bending angles in three different projections: perpendicular to the internal (Arrow a), the external (Arrow b) and the middle arc (Arrow c). Images were taken under different magnifications to show through the bending angle and the position of the pattern as well. Using an open source image analysis software, ImageJ (Version 1.49k 9 November 2014), two sides and the largest and smallest angles of the tetragons were measured. The wire tetragons demonstrated characteristics of a parallelogram its opposing side lengths and angles were roughly equal.
Table 1. Specification of samples.
Sample | Diameter (mm) | Wire material | Marker wire material | Mesh density (pores/mm2) | Number of wires | Number of marker wires |
A | 4.5 | CoCr alloy | 92% Pt 8% W | 20-32 | 72 | 12 |
B | 5 | CoCr alloy | 92% Pt 8% W | 20-32 | 72 | 12 |
C | 5 | 75% CoCr alloy and 25% Pt-W | 20-50 | 48 | - |
To determine MSA, sharp sections of the images were selected, representing the wire pattern of the specific sample. These images were analysed with ImageJ, the area of the whole section was measured and based on the principle of separation of lighter and darker areas, the program measured the percentage of the metallic surface area corresponding to the MSA value in the observed position. This eliminates inconsistencies caused by areas out of focus and selection of the area analysed, as the MSA of each area was measured independently.
3. Results
Table 2-4 shows the average opening angle and MSA at the three examined projections of the bending angles. The biggest opening angles were found at the internal projection in all but three measuring points. It was also observed that with increasing bending angle, the opening angles increasingly differed and gradually converged to the non-bended value as expected. The lowest opening angles were measured on sample “B”, the biggest ones on sample “C”.
No similar trend was found for MSA. However, it was noted that the ratio of metal covered area in the external projections was higher than in the middle projections. The difference between the calculated values (both MSA and the angles measured) in different positions became smaller by increasing the bending angle and also converged to the value of non-bended state like the opening angle.
Table 2. Sample “A” MSA values in the observed projections.
Projection | Angles (°) | MSA (%) | Projection | Angles (°) | MSA (%) |
0° | 90.67 | 33 | |||
30° | 90° | ||||
external | 57.23 | 34.4 | external | 75.57 | 33.0 |
middle | 106.61 | 29.2 | middle | 92.50 | 17.0 |
internal | 137.49 | 32.7 | internal | 102.72 | 38.3 |
45° | 120° | ||||
external | 39.80 | 43.7 | external | 72.24 | 32.3 |
middle | 118.07 | 30.0 | middle | 76.39 | 30.3 |
internal | 139.34 | 37.5 | internal | 77.80 | 30.1 |
60° | 135° | ||||
external | 76.76 | 36.8 | external | 100.90 | 36.4 |
middle | 100.75 | 30.9 | middle | 111.27 | 37.0 |
internal | 130.89 | 41.1 | internal | 108.69 | 38.9 |
Table 3. Sample “B” MSA values in the observed projections.
Projection | Angles (°) | MSA (%) | Projection | Angles (°) | MSA (%) |
0° | 102.95 | 35.9 | |||
30° | 90° | ||||
external | 54.68 | 40.8 | external | 78.10 | 35.6 |
middle | 99.99 | 29.3 | middle | 114.78 | 33.7 |
internal | 152.90 | 49.9 | internal | 145.75 | 57.3 |
45° | 120° | ||||
external | 34.76 | 52.3 | external | 64.66 | 33.9 |
middle | 118.37 | 26.2 | middle | 97.04 | 31.5 |
internal | 148.39 | 50.9 | internal | 106.87 | 33.6 |
60° | 135° | ||||
external | 54.42 | 30.4 | external | 78.94 | 36.1 |
middle | 98.92 | 21.4 | middle | 95.87 | 35.1 |
internal | 157.58 | 51.4 | internal | 117.65 | 35.9 |
Table 4. Sample “C” MSA values in the observed projections.
Projection | Angles (°) | MSA (%) | Projection | Angles (°) | MSA (%) |
0° | 153.638 | 49.5 | |||
30° | 90° | ||||
external | 121.28 | 20.7 | external | 138.14 | 29.5 |
middle | 131.98 | 27.3 | middle | 150.29 | 35.2 |
internal | 159.14 | 48.6 | internal | 160.04 | 61.1 |
45° | 120° | ||||
external | 131.76 | 32.7 | external | 141.57 | 28.1 |
middle | 150.90 | 31.5 | middle | 152.10 | 31.4 |
internal | 162.85 | 55.6 | internal | 152.42 | 37.2 |
60° | 135° | ||||
external | 133.81 | 25.5 | external | 151.40 | 31.3 |
middle | 142.38 | 30.1 | middle | 141.67 | 26.1 |
internal | 161.74 | 57.9 | internal | 146.33 | 28.4 |
It was seen that the MSA was higher for extremely high (over 140°) and extremely small (under 40°) opening angle values. The previous conclusion that the wire mesh consists of parallelograms also confirms the obtained results since opposite angles in a parallelogram are the same and the sum of internal angles is 360°. Consequently, the decrease of the observed angle caused another angle to increase. Hence, after a certain angle, the same parallelogram was obtained but rotated by 90° and the function of MSA at the opening angle showed a parabolic nature.
4. Conclusion
The effect of flow diverters for treatment of intracranial aneurysms is thought to be related to the MSA value. Due to frequent tortuosity of cerebral vascular segments, flow diverters are often implanted in curved sections. Our research provide additional useful information about wire mesh pattern change of cerebrovascular flow diverters caused by vessel geometry. The required MSA value for efficacy based on the manufacturers’ recommendations must be 30-55%. In our test method the devices could not provide this rate in many bending angles and projections, the lowest measured values of 17-21% mean a relevant deviation at all three samples. There is no standard procedure to determine MSA value of FDs, however the method used in this study provide a consistent solution. The impact of vessel geometry may significantly influence the MSA and subsequently the efficacy of the device. This study demonstrated that as the bending angle increased, the opening angle also increased in all three cases. The opening angle of parallelograms of the pattern might be an ideal starting point for real coverage indicator. It would be worth to calculate a function between the opening angles in non-bended state and in each bending angles, so we could determine suitability of the flow diverter implantation in curved vessel.
Acknowledgements
This work is connected to KTIA_NAP_13-1-2013-0001 Hungarian Brain Research Program.
References
[1] M. M. Y. Tse, B. Yan, R. J. Dowling, P. J. Mitchell. “Current Status of Pipeline Embolization Device in the Treatment of Intracranial Aneurysms: A Review,” in World Neurosurgery. 2013, 80, vol. 6, pp. 829-835. View Article
[2] F. Briganti, M. Napoli, G. Leone, M. Marseglia, G. Mariniello, F. Caranci, F. Tortora, F. Maiuri, “Treatment of intracranial aneurysms by flow diverter devices: Long-term results from a single center,” in European Journal of Radiology, 2014, vol. 83, pp. 1683–1690. View Article
[3] S. B. Murthy, S. Shah, C. P. Venkatasubba Rao, E. M. Bershad, J. I. Suarez, “Treatment of unruptured intracranial aneurysms with the pipeline embolization device,” in Journal of Clinical Neuroscience, 2014, vol. 21, pp. 6–11. View Article
[4] L. Pierot, “Flow diverter stents in the treatment of intracranial aneurysms: Where are we?,” in Journal of Neuroradiology, 2011, vol. 38, pp. 40-46. View Article
[5] P. K. Nelson, P. Lylyk, I. Szikora, S. G. Wetzel, I. Wanke, D. Fiorella, “The Pipeline embolization device for the Intracranial Treatment of Aneurysms trial” in American Journal of Neuroradiology, 2011, vol. 32, pp. 34-40. View Article
[6] G. K. C. Wong, M. C. L. Kwan, R. Y. T. Ng, S. C. H. Yu, W. S. Poon, “Flow diverters for treatment of intracranial aneurysms: Current status and ongoing clinical trials,” in Journal of Clinical Neuroscience, 2011, vol. 18, pp. 737–740. View Article
[7] M. Shapiro, E. Raz, T. Becske, P.K. Nelson (2014). “Variable Porosity of the Pipeline Embolization Device in Straight and Curved Vessels: A Guide for Optimal Deployment Strategy,” in American Journal of Neuroradiology, 2014, vol. 35, pp. 727–33. View Article