Volume 3 - Year 2016 - Pages 43-47
DOI: 10.11159/jbeb.2016.008
Pegylated Curcumin with Gold Nanoparticles: Antimicrobial Agent Evaluation
Daniella dos Santos Courrol1,
Bruna Henrique Teixeira2, Camila Bueno Pacheco Pereira1, Marcia Regina Franzolin1, Lilia Coronato Courrol2
1Instituto Butantan, Laboratório de Bacteriologia, São Paulo, SP, Brazil.
2Universidade Federal de São Paulo, Laboratório de Lasers e óptica Biomédica Aplicada, Departamento de Ciências Exatas e da Terra, Diadema, SP - Brazil
lccourrol@gmail.com
Abstract - Curcumin is a polyphenol derived from the Curcuma longa plant. Curcumin exhibits a variety of therapeutic properties, including antioxidant, analgesic, anti-inflammatory, anticancer and antiseptic activities. Curcumin acts as photosensitizer in photodynamic therapy (PDT), which is an alternative way to kill pathogenic microorganisms. The use of gold nanoparticles (AuNPs) as the vehicle for curcumin delivery increases photodynamic ability. Therefore, the aim the present study is to investigate the effect of the photodynamic action of LED-activated curcumin gold nanoparticles, on cell viability of Staphylococcus aureus (Sa), Staphylococcus epidermidis (Se), Pseudomonas aeruginosa (Pa), Escherichia coli (Ec), Citrobacter freundii (Cf) and Klebsiella pneumoniae (Kp). To prepare Curcumin gold nanoparticles (Curc:AuNps) solutions, 3.2 mg of HAuCl4 was mixed with 1.5 mg Curcumin and Polyethylene glycol (PEG) in Mili-Q water. This solution was exposed to light from a mercury metal halide lamp. The resulting solutions were characterized by UV-Vis and transmission electron microscopy (TEM). The in vitro antibacterial activity of Curc:AuNps was evaluated against Sa, Se, Pa, Ec, Cf and Kp using the broth dilution assay. The effects of exposure to yellow LED light with ~38 J/cm2 fluence and variations in the Curc:AuNPs concentrations (38, 50, 75 and 100% dilutions) were studied. The presence of a surface plasmon resonance band at ~560 nm indicated the formation of spherical gold nanoparticles. In the presence of PEG, the solution color changed some minutes after the mixing of reagents, and solution size homogeneity was improved with the mercury metal halide lamp illumination. TEM analyses showed ~17±2 nm nanoparticles. The results indicate that PDT with curcumin gold nanoparticles inhibits the development of Gram positive and Gram negative bacteria. This study revealed antibacterial probability of inhibition >80% with 32 mM of Curc:AuNPs and light against Sa, Se, Cf and Kp strains after 18 hours of incubation. NOVA/Tukey's tests were conducted to compare groups curcumin/Curc:AuNPs, with and without photodynamic excitation.
Keywords: Gold nanoparticles, curcumin, photodynamic therapy, antibacterial.
© Copyright 2016 Authors This is an Open Access article published under the Creative Commons Attribution License terms. Unrestricted use, distribution, and reproduction in any medium are permitted, provided the original work is properly cited.
Date Received: 2016-04-28
Date Accepted: 2016-08-28
Date Published: 2016-12-20
1. Introduction
Curcumin (diferuloylmethane), is a polyphenol that accumulates in the rhizome of Curcuma longa, can reduce the risk of heart disease, high blood pressure and cognitive decline1-8. Additionally, due to its capabilities to regulated important transcription factors, protein kinases, cytokines, adhesion molecules and redox status, curcumin can serve as an anti-inflammatory, anti-oxidant, anti-angiogenic, antimicrobial and antineoplasic agent9.
Despite progress in development of antibacterial agents, there are still special needs to find new antibacterial agents due to development of multidrug resistant bacteria. Curcumin has been shown to inhibit cellular proteins FtsZ10 and sortase A11, thereby interrupting cytokinesis and cellular adhesion, and interfere with biofilm formation12. Though curcumin exhibits broad antimicrobial activity, some data suggest that Gram-positive species are more susceptible than Gram-negatives1, 9. In adition, Antibiotics are sometimes associated with adverse effects on the host including hypersensitivity, immune-suppression and allergic reactions. Curcumin described as prebiotic, which have beneficial effects on human health.
In this paper the antibacterial properties of curcumin will be evaluated and alternatives to increase these properties will be studied: the synthesis of gold nanoparticles using curcumin as reductor agent and uses of light to stimulate photodynamic properties of curcumin.
Studies shown that the bioavailability of curcumin can be increased with its conjugation on the surface of gold nanoparticles. Metal nanoparticles can be produced by chemical reduction methods, physical methods as photoreduction13, microwave dielectric heating14, ultrasonic irradiation15, and radiolysis16, and biological routes 17. Electromagnetic radiation is a green alternative to the synthesis of nanoparticles, since it is free of environmentally negative effects, such as use of toxic solvents or aggressive reducers18. The use of light in the synthesis of nanoparticles have emerged in 1999 with Zhou et al. 19, when shape-controlled gold nanoparticles were synthesized using ultraviolet irradiation technique at room temperature. Dong et al. 20 used sunlight to synthesize nanoparticles of gold in suspension. Tomita et al. obtained silver nanoparticles with Tryptophan and light and observed its lethal effect against bacteria 21. Mao et al.22 obtained size and shape deviations of <6% of the gold nanoparticles under optimal ultraviolet irradiation and chemical conditions.
Gangwar et al. in 201223, reported the formation of curcumin conjugated with gold nanoparticles using Polyvinyl pyrrolidone which has been used as drug carrier for various drugs. In 2013 Dheeraj et al. synthesized the gold nanoparticles (AuNPs) by direct reduction of chloroauric acid (HAuCl4) using curcumin in an aqueous phase without the use of any other reducing agents. Sreelakshm et al. 24 synthesized gold nanoparticles using turmeric rhizome extract, curcumin and curcumin glycoside acting as reducing and stabilizing agents at room temperature. They observed that the carbonyl group of enolic curcumin helps in reduction as well as stabilization of gold nanoparticles. Shlar et al. 23, 25 prepared water-dispersible curcumin nanoparticles by bottom-up antisolvent precipitation approach and observed a minimal inhibitory concentration of 400 or 500µM toward Escherichia coli.
Curcumin also acts as photosensitizer in Photodynamic therapy (PDT) [14], an alternative way to kill pathogenic microorganisms. In PDT, photosensitizers, are activated by visible light to produce cytotoxic reactive oxygen species (ROS), which induce lethal damages on target cells and tissues [5-8]. In comparison to conventional antibiotic treatment, PDT has unique advantages of dual specificity in targeting eradication of pathogenic microorganisms: preferential absorption of pathogenic microorganisms and target lesion irradiated from laser or visible light. Furthermore, the resistance of bacteria to PDT is very unlikely because of the nonspecific damage of PDT on bacteria [12]. So, PDT has shown potential promise in eradicating pathogenic microorganisms and treating infectious diseases. The use of AuNPs as the vehicle for curcumin delivery represents a promising approach, especially if the immobilization of curcumin on the particle surface be better for ROS formation. Therefore, in the present study our aim is also to investigate the effect of photodynamic action of LED-activated curcumin on cell viability of Staphylococcus aureus, Staphylococcus epidermidis, Pseudomonas aeruginosa, Escherichia coli, Citrobacter freundii and Klebsiella pneumoniae, in the presence of gold nanoparticles.
2. Materials and Methods
2.1. Gold nanoparticles generation and evaluation
Chloroauric acid, HAuCl4 99%, CAS 27988-77-8) (~1.5mg), Polyethylene Glycol (PEG) 10000 (10mg/m) and Curcumin (3.2 mg) from Curcuma Longa (CAS 458-37-7) were purchased from Sigma-Aldrich. No other chemical reagents were used in the synthesis. The gold nanoparticles (CurcAuNPs) were produced by mixing the reagents in 100mL of miliQ water solution, followed by vigorous stirring and illumination with a metal halide bulb lamp (Leica EL6000), not focused and placed 10 cm from the sample reservoir for 15 min.
UV-vis absorption spectra were recorded by a Shimadzu Multispec-1501 UV-vis spectrophotometer using 10-mm quartz cells.
The morphology of the curcumin nanoparticles (Curc:AuNPs) was determined by transmission electron microscopy using a LEO 906E instrument from Zeiss, Germany (6 µA and 80 kV). For the measurements, a drop of gold nanoparticles (5 μL) dispersed in bi-deionized water was placed onto a carbon-coated copper grid. The excess liquid was removed using a paper wick, and the deposit was dried in air for 5 min prior to imaging. The images were captured by a Megaview III camera and processed using the iTEM universal TEM imaging platform (Olympus Soft Imaging Solutions GmbH, Germany).
2.2. Broth Microdilution Assay for the determination of antimicrobial activity
The microorganisms used in this assay were: Staphylococcus aureus American Type Culture Collection (ATCC) 25923, Staphylococcus epidermidis (clinical sample), Pseudomonas aeruginosa ATCC 27853, Escherichia coli ATCC 25922, and the clinical samples of Citrobacter freundii and Klebsiella pneumoniae.
The antimicrobial activity of the tested substances was determined by 2-fold serial broth microdilution in culture medium in 96-well plates. The assays were conducted according to the guidelines of the National Committee for Clinical Laboratory Standards (NCCLS. Performance Standards for Antimicrobial Susceptibility Testing; Twenty-Fifty Informational Supplement. (NCCLS, 2012), for determination of Minimal Inhibitory Concentration (MIC). Aliquots of 50 µl of Mueller-Hinton broth (Difco) with bacterial inoculum adjusted to approximately 106 CFU/ml was applied in the microplates containing 50 µl of the diluted solutions, resulting in a final volume of 100 µl with 104 CFU/well. A positive growth control containing only culture medium and the strains under study was also prepared. The 100 µL MH liquid culture medium was used as negative control in the study. The nanoparticles concentrations used were: 38% (11.3µg/mL), 50%(15.0µg/mL), 75% (22.5µg/mL) and 100% (30.0µg/mL).
After inoculation, the plates were incubated at 37°C for 18 h before the results were recorded. After this time, the turbidity of the cultures was measured in an ELISA reader (Multiskan®EX (Thermo Isher Scientific, EUA) at 595 nm to assess bacterial growth. The results were expressed as inhibition percentage of optical density (OD) against a control; this control was obtained in each situation by measuring the OD of the microorganisms introduced into the plate in the absence of solutions. All of the tests were performed in triplicate.
3. Results and Discussion
Applying broth microdilution testing to a number of bacterial strains provides an estimate of the concentration that inhibits bacterial isolates and can indicate shifts in the susceptibility of bacterial populations to gold nanoparticles. This antimicrobial activity assay is therefore often the starting point for larger preclinical evaluations of novel antimicrobial agents.
To pursuit the broth microdilution testing, initially the gold nanoparticles were synthesized to obtain the right concentration, size and stability. Photodynamic effectivity was evaluated comparing the bacterial inhibition results obtained with and without light incubation.
3.1. Synthesis of Curcumin gold nanoparticles (CurcAuNPs)
The absorption spectrum of the solution measured after illumination by the Hg for 15 minutes is shown in Figure 1. As can be seen in this figure, the irradiated solution exhibits the plasmon resonance band typical for Au nanoparticles centered at ~562 nm.
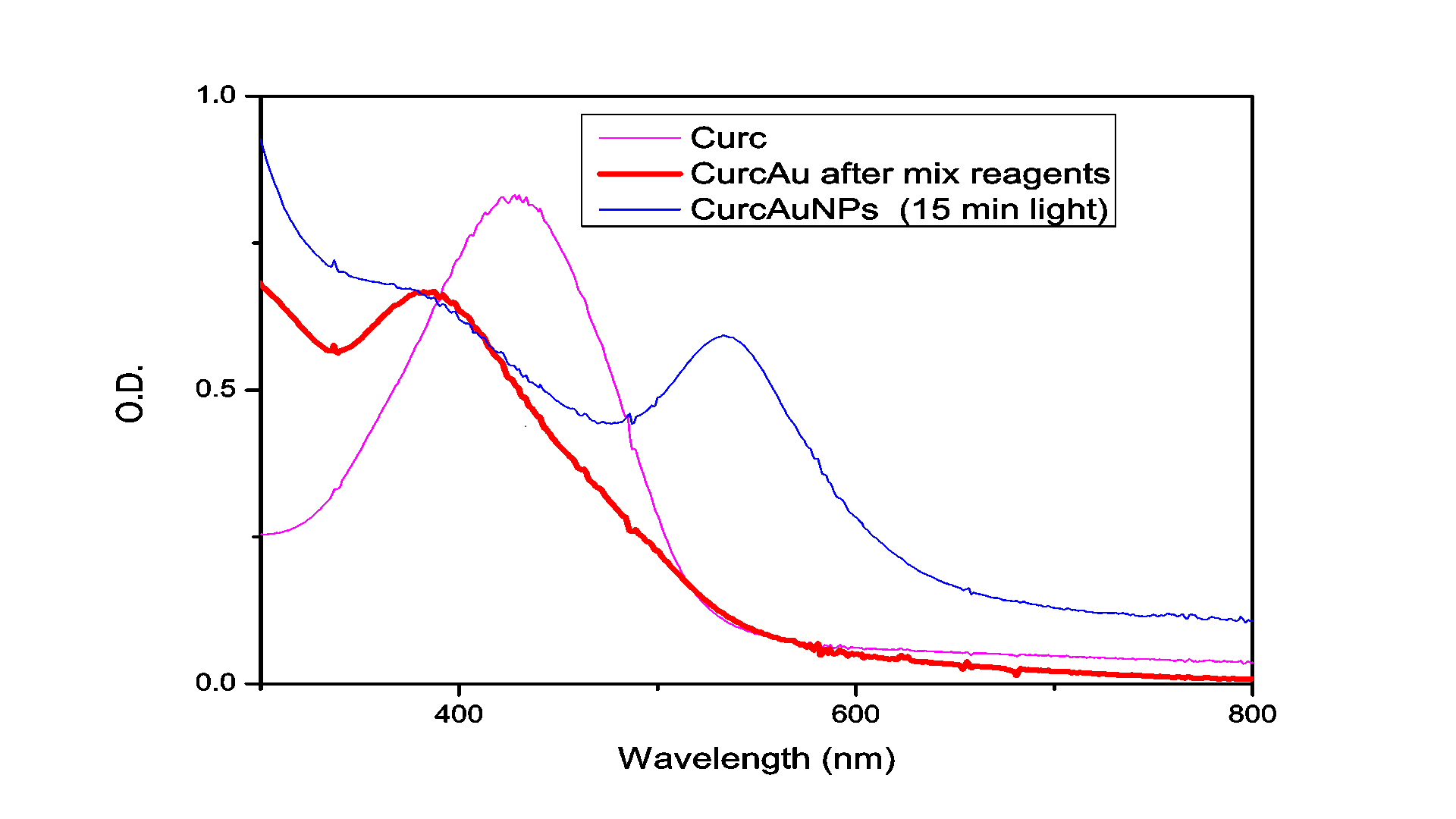
TEM was used to characterize the size, shape and morphology of the synthesized CurcAuNPs. The TEM images of synthesized nanoparticles are shown in Figure 2. The morphology of CurcAuNPs is nearly spherical. The CurcAuNPs sizes ranged from 15 to 18 nm, as observed in figure 2.
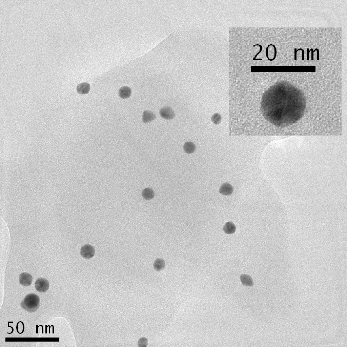
3.2. Antimicrobial activity
A study on the sensitivity of bacteria to exposure to CurcAuNPs was performed. This study was achieved according to the microdilution test26 and provided results for the inhibition of growth of certain microorganisms.
The microdilution test provided quantitative results concerning the inhibition of bacterial growth, as obtained by reading the plates in a spectrophotometer. The data from the experiments performed in triplicate are shown in Figures 3 referring to the incubation periods of 18 hours, without and with PDT, respectively.
The results indicate that the gold nanoparticles strongly inhibited the development of Gram positive and Gram negative bacteria. After 18 hours of incubation with CurcAuNPs, inhibition of all of the tested strains where higher than incubation for concentrations higher that 15 µg/mL. For S. aureus, the inhibition of which in the presence of the highest concentration of the antimicrobial agent was close to 98% at 30 µg/mL. For S. epidermidis concentrations of 22.5 and 30 µg/mL of CurcAuNPs induces a change in absorption spectra of bacteria probably due to aptoptosis. The E. coli was the unique strain that showed good response for PDT and 30 µg/mL of CurcAuNPs. Other light irradiation conditions must be studied to understand the PDT effect.
According to the literature, the interaction of gold nanoparticles is that the nanoparticles are capable of penetrating the inner bacterial cell can interact with DNA molecules through the phosphorus groups, leading to loss of the capacity for cell replication.
AuNPs mainly exert their antibacterial action in three ways27: 1) by changing membrane potential and inhibiting adenine triphosphate (ATP) synthase activities to lower the level of ATP, activating metabolic pathways that generate anabolic reactions. The ATP-dependent mechanism essentially consists of pumping the antimicrobial ions that are present in the cytoplasm to the extracellular medium. 2) by inhibiting the subunit of ribosome for tRNA (transfer ribonucleic acid) binding, generating a blockade of the vital reactions in bacteria. 3) Some authors recently suggested that the inhibitory action occurs due to free radicals derived from the particle surface that are capable of damaging the cell membrane. The efflux pumps are recognized as one of the main forms of antibiotic resistance and metal ion mechanism. In the presence of a sensitizer, ROS (reactive oxygen species)-related process occurs summated to the other processes.
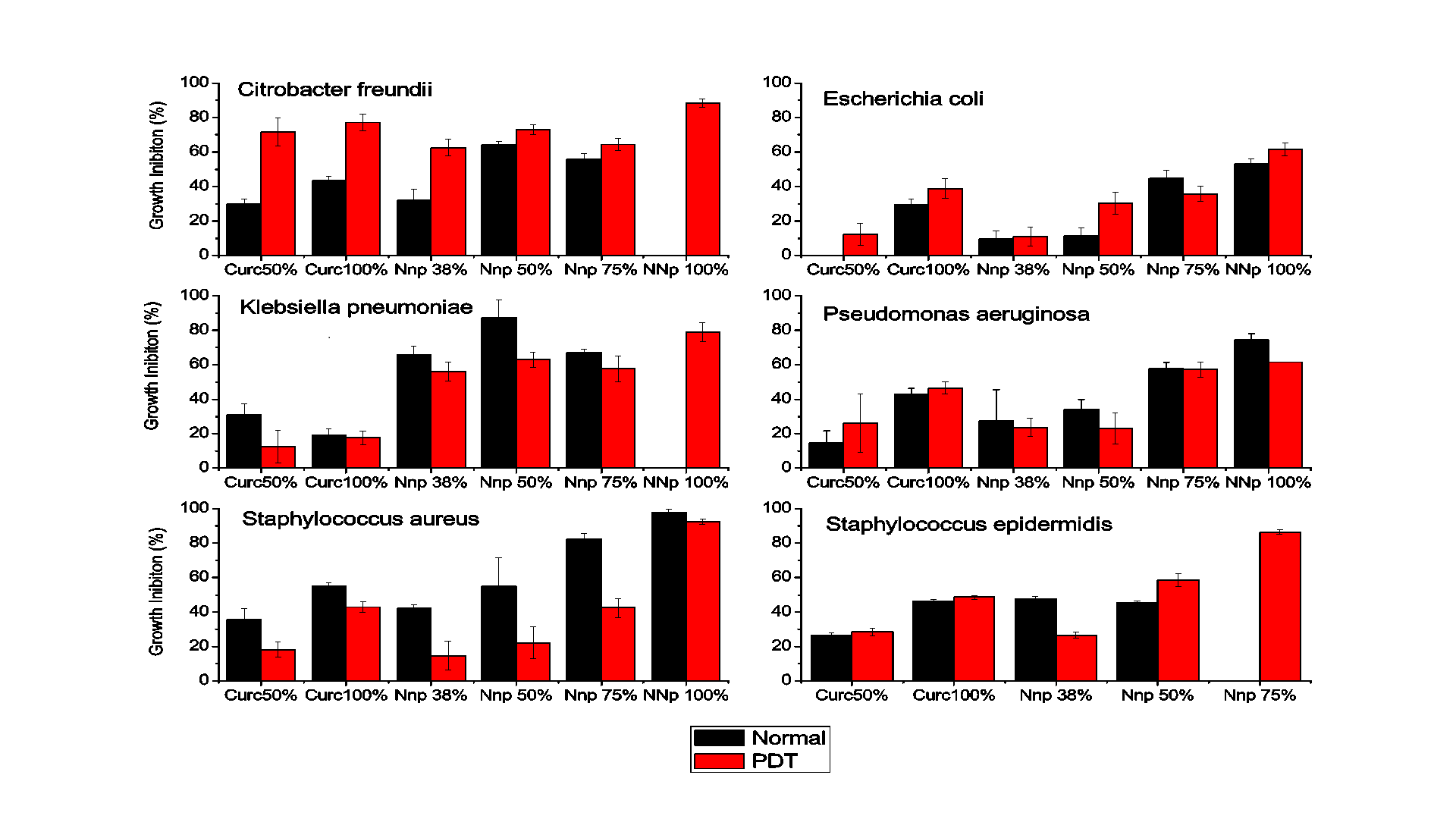
4. Conclusion
In this paper, Curcumin gold nanoparticles were synthesized using a green chemistry method based on Curcumin, PEG and light. The preparation conditions were optimized using the results of absorption spectroscopy, and the antimicrobial effect of the resulting nanoparticles was tested.
The antimicrobial sensitivity test performed using the broth microdilution method provided quantitative data on the inhibitory effect of gold nanoparticles on microorganisms, which, in concentrations greater than 22.5 µg/mL, revealed that the inhibition was close to 50% for period of 18 hours. Thus, the gold nanoparticles synthesized based on the concepts of the green chemistry methodology exhibited promising results regarding the development of the inhibitory action to different bacteria species. In general PDT, in the used illumination conditions, does not presented good improvements.
Acknowledgements
The National Council for Scientific and Technological Development (CNPq) is acknowledged for their financial support and Fapesp (grant 2014/06960-9), and Flávia Rodrigues de Oliveira Silva is acknowledged for providing the electron microscope support. Daniella dos Santos Courrol is fellowship of PAP, Secretariat of Health fellowship.
References
[1] Y. Wang, Z. Lu, H. Wu and F. Lv, International Journal of Food Microbiology, vol. 136, pp. 71-74, 2009. View Article
[2] R. L. Thangapazham, S. Sharad and R. K. Maheshwari, Biofactors, vol. 39, pp. 141-149, 2013. View Article
[3] C. Syng-ai, A. L. Kumari and A. Khar, Molecular Cancer Therapeutics, vol. 3, pp. 1101-1108, 2004. View Article
[4] R. A. Sharma, A. J. Gescher and W. P. Steward, European Journal of Cancer, vol. 41, pp. 1955-1968, 2005. View Article
[5] T. A. Nguyen and A. J. Friedman, Journal of Drugs in Dermatology, vol. 12, pp. 1131-1137, 2013. View Article
[6] J. J. Johnson and H. Mukhtar, Cancer Letters, vol. 255, pp. 170-181, 2007. View Article
[7] P. Hu, P. Huang and M. W. Chen, Archives of Oral Biology, vol. 58, pp. 1343-1348, 2013. View Article
[8] S. C. Gupta, S. Patchva and B. B. Aggarwal, Aaps Journal, vol. 15, pp. 195-218, 2013. View Article
[9] R. K. Basniwal, H. S. Buttar, V. K. Jain and N. Jain, Journal of Agricultural and Food Chemistry, vol. 59, pp. 2056-2061, 2011. View Article
[10] D. Rai, J. K. Singh, N. Roy and D. Panda, Biochemical Journal, vol. 410, pp. 147-155, 2008. View Article
[11] B. S. Park, J. G. Kim, M. R. Kim, S. E. Lee, G. R. Takeoka, K. B. Oh and J. H. Kim, Journal of Agricultural and Food Chemistry, vol. 53, pp. 9005-9009, 2005. View Article
[12] T. Rudrappa and H. P. Bais, Journal of Agricultural and Food Chemistry, vol. 56, pp. 1955-1962, 2008. View Article
[13] A. Callegari, D. Tonti and M. Chergui, Nano Letters, vol. 3, pp. 1565-1568, 2003. View Article
[14] S. Horikoshi, H. Abe, K. Torigoe, M. Abe and N. Serpone, Nanoscale, vol. 2, pp. 1441-1447, 2010. View Article
[15] R. Gottesman, S. Shukla, N. Perkas, L. A. Solovyov, Y. Nitzan and A. Gedanken, Langmuir, vol. 27, pp. 720-726, 2011. View Article
[16] H. T. Tung, I. G. Chen, I. M. Kempson, J. M. Song, Y. F. Liu, P. W. Chen, W. S. Hwang and Y. Hwu, ACS Appl Mater Interfaces, vol. 4, pp. 5930-5935, 2012. View Article
[17] M. Roni, K. Murugan, C. Panneerselvam, J. Subramaniam, M. Nicoletti, P. Madhiyazhagan, D. Dinesh, U. Suresh, H. F. Khater, H. Wei, A. Canale, A. A. Alarfaj, M. A. Munusamy, A. Higuchi and G. Benelli, Ecotoxicol Environ Saf, vol. 121, pp. 31-38, 2015. View Article
[18] N. Li, P. Zhao and D. Astruc, Angewandte Chemie-International Edition, vol. 53, pp. 1756-1789, 2014. View Article
[19] Y. Zhou, C. Y. Wang, Y. R. Zhu and Z. Y. Chen, Chemistry of Materials, vol. 11, pp. 2310-+, 1999. View Article
[20] S. Dong, C. Tang, H. Zhou and H. Z. Zhao, Gold Bulletin, vol. 37, pp. 187-195, 2004. View Article
[21] R. J. Tomita, R. A. de Matos, M. A. Vallim and L. C. Courrol, Journal of Photochemistry and Photobiology B-Biology, vol. 140, pp. 157-162, 2014. View Article
[22] X. Mao, J. Kwon, H. Zhou and J. Lee, Current Applied Physics, vol. 15, pp. 1100-1105, 2015. View Article
[23] R. K. Gangwar, V. A. Dhumale, D. Kumari, U. T. Nakate, S. W. Gosavi, R. B. Sharma, S. N. Kale and S. Datar, Materials Science & Engineering C-Materials for Biological Applications, vol. 32, pp. 2659-2663, 2012. View Article
[24] C. Sreelakshmi, N. Goel, K. K. R. Datta, A. Addlagatta, R. Ummanni and B. V. S. Reddy, Nanoscience and Nanotechnology Letters, vol. 5, pp. 1258-1265, 2013. View Article
[25] I. Shlar, E. Poverenov, Y. Vinokur, B. Horev, S. Droby and V. Rodov, Nano-Micro Letters, vol. 7, pp. 68-79, 2015. View Article
[26] P. S. f. A. S. Testing and T.-F. I. Supplement, NCCLS document M100-S25, Wayne, PA: National Committee of Clinical Laboratory Standards, 2012. View Book
[27] Y. Cui, Y. Zhao, Y. Tian, W. Zhang, X. Lu and X. Jiang, Biomaterials, vol. 33, pp. 2327-2333, 2012. View Article