Volume 3 - Year 2016 - Pages 1-3
DOI: 10.11159/jbeb.2016.001
Mechanical Simulator of the Small Intestine for In-Vitro Practice with Endoscopic Devices
Oren M. Rotman1, Uri Zaretsky1, Yaniv Birnboim2, Amit Pascal2, Shmuel Einav1
1The Department of Biomedical Engineering, Tel-Aviv University
Ramat Aviv, Tel Aviv, Israel, 6997801
orenrotman1@gmail.com; uriza@eng.tau.ac.il; einav@eng.tau.ac.il
2Given Imaging Ltd.
2 Hacarmel St., New Industrial Park, POB 258, Yoqneam, Israel, 2069200
Yaniv.Birnboim@covidien.com; Amit.Pascal@covidien.com
Abstract - The small intestine (SI) is a mechanically active tube-like organ that actuates peristaltic constriction motion. The motion assists capsule endoscopy products to passively travel along the SI tract. The goal of the present study was to design a mechanical simulator of the small intestine. Having such a simulator would allow effective testing of new capsule endoscopic devices, as well as offline practice with endoscopic and other devices. The simulator, loaded with ex-vivo swine SI, was functionally validated using two ingestible capsule products: PillCam® SB3 and SmartPill® (Given Imaging, Ltd.). Excellent agreement was found in comparison to in-vivo recordings, showing the great potential of the simulator.
Keywords: Bowel, Gut, Peristaltic motion, Capsule endoscopy, Pillcam
© Copyright 2016 Authors - This is an Open Access article published under the Creative Commons Attribution License terms. Unrestricted use, distribution, and reproduction in any medium are permitted, provided the original work is properly cited.
Date Received: 2015-10-05
Date Accepted: 2015-02-15
Date Published: 2016-05-02
1. Introduction
The small intestine (SI) is a mechanically active tube-like organ, where most of the digestion and absorption of nutrients takes place. A peristaltic constriction motion of the SI tube allows a slow internal motion of the digested food toward the colon. Based on that motion, capsule endoscopy products passively travel along the SI tract and allow its imaging with minimal intervention. The capsule size is in the order of a large pill and usually contains a miniature camera, light source and imaging pre-processing technology. The primary use of capsule endoscopy is to examine areas of the small intestine that cannot be reached by other technologies, such as colonoscopy or esophagogastroduodenoscopy (EGD). The technique was approved by the U.S. Food and Drug Administration (FDA) in 2001. Ingestible capsule products, in addition to image capturing, can measure pH, temperature, and pressure [1], and even utilize x-ray 3D imaging of the gastrointestinal tract [2], [3].
Very few in-vitro simulators of the SI have been introduced in the past. Most of them simulated the chemical effects of the SI [4], [5], while only one simulated the mechanical effects [6]. None of these, however, were sufficient for capsule endoscopy or other endoscopic product testing. Our in-vitro SI motility simulator minimizes the costly and time consuming animal tests. . It allows easier and quicker testing of new ingestible capsule products even during early R&D stages. It also facilitates training of clinicians with endoscopic devices in realistic environment.
2. Design
The SI simulator is based on 24 circular inflating units of about 1 cm width each that are assembled consecutively (Figure 1). Pressurized air is supplied to each unit and causes circular inflation and orifice closing. The inflation units are connected separately to an inflation controller which sequentially inflates the units in a peristaltic-like motion. Both peristaltic velocity and intensity can be managed manually by the operator, and the number of simultaneously inflated units (compression width) can be altered. The inflation intensity is altered by a pneumatic regulator, while the rate by an electronic one. The SI loop itself is an independent elastic tube, about 25 cm long, which is concentrically loaded inside the inflating units. It can be made by an explanted SI segment, or by any elastic tube with similar size (e.g. Penrose drainage tube). The SI loop which is loaded in the simulator is connected to an open flow loop, so that peristaltic compression of the inflation units induces a peristaltic motion and flow in the SI loop itself. The working fluid can be water, bile, or any other solution that is compatible with the components of the simulator. Introduction of air bubbles into the flow loop is also controllable by introduction of pressurized air into a reservoir at the entrance to the flow loop.
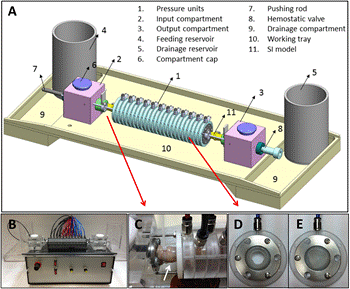
3. Capsule Testing
The functional operation of the simulator was validated using two capsule devices: PillCam® SB3 (Given Imaging Ltd., Yokneam, Israel) for qualitative visualization purposes of the SI motility, and SmartPill® (Given Imaging Ltd., Yokneam, Israel) for pressure measurements of the peristaltic motion. The PillCam® SB3 capsule was tested on two SI loop models: 1) Penrose drainage tube of 15 mm diameter and 1 mm thickness (Thai Centri Co., Ltd., Kratumban, Thailand); 2) section of swine SI. For the second model, a preservation procedure was applied before the ex vivo testing. The SmartPill® was tested on preserved SI tissue only. The date obtained from measurements performed in the SI simulator were compared to archive in-vivo recordings from human subjects.
Preservation of the SI tissue comprised of soaking the SI segments in 1% Glutaraldehyde solution (Sigma-Aldrich Israel Ltd., Rehovot, Israel) for 1 hour, followed by double rinsing with PBS (BI Beit Haemek Ltd., Beit Haemek, Israel). The samples were then stored refrigerated at 4oC available for testing. This mild preservation process kept the SI tissue for about 30 days, with minor impairment of the mechanical properties of a fresh tissue.
Data acquisition: the PillCam® SB3 uses RF transmission to transmit about two images per second to a data recording device that is ordinarily attached to a belt worn around the patient’s waist or to sensor arrays on the patient’s chest. The images are then downloaded to a computer for review and analysis. In this study, sensor arrays were used and placed several centimetres outside the inflating balloon assembly. Data acquisition from the SmartPill® is identical to the PillCam, with two pressure readings per second.
4. Results
The experiments demonstrated that the proposed simulator ensured small intestine model contractions and capsule movement similar to that in-vivo. The transit speed used for the capsules was about 0.5 cm/s, with fully controllable speed range of zero to several centimetres per second. Images taken by the PillCam® SB3 capsule indicated excellent similarity of the SI internal wall to that taken in-vivo (Figure 2), when both the contraction motion as well as the architecture of the SI wall (villi structure) were maintained. The pressure recordings of the SmartPill® showed similarity in the pressure wave morphology, yet with higher baseline pressure in the SI simulator (about 30 mmHg, versus 0 mmHg in-vivo). This elevated offset pressure, and the pressure wave amplitude, were accounted to the water column applied in the reservoirs, and the inflation pressure respectively. The typical pressure amplitude of SI contractions in-vivo in our recordings from swines was varied in the range of about 5 to 65 mmHg (results not shown). Previous measurements in-vivo in human subjects reported on peak contraction amplitude of 12.94±7.22 mmHg [7]. Stokes, et al. [8], which investigated the gastrointestinal tract of ponies, have reported on average maximum SI contraction pressure of 81.8 mmHg. As expected, the peristaltic motion frequency was much more uniform in the simulator than in-vivo. Since the peristaltic motion frequency and intensity (pressure) are fully controlled by the operator (ranges: 0-180 contractions/minute, and 0-250 mmHg), the setup can be easily adjusted to mimic a variety of in vivo scenarios.
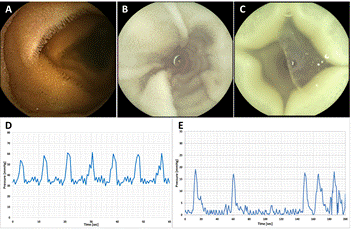
5. Future Work
The presented version of the SI simulator comprised of a mechanically-based inflation controller which synchronized the action of the inflation units, and subsequent peristaltic motion. Advances in the next generation will include a digital inflation controller that allows a better monitoring of the peristaltic contractions, and applying in-vivo pressure recordings as an input, for even more realistic simulations.
6. Conclusion
Overall, the SI simulator showed great potential for in-vitro testing of capsule endoscopic products, enables reduction in number of animal tests, and improves the R&D procedure.
References
[1] B. Kuo, R. W. Mccallum, K. L. Koch, M. D. Sitrin, J. M. Wo, W. D. Chey, W. L. Hasler, J. M. Lackner, L. A. Katz, J. R. Semler, G. . E. Wilding and H. P. Parkman, "Comparison of gastric emptying of a nondigestible capsule to a radiolabelled meal in healthy and gastroparetic subjects," Alimentary Pharmacology & Therapeutics, vol. 27, no. 2, p. 186–196, 2008. View Article
[2] M. Moshkowitz and N. Arber, "Safety and Proof of Concept Demonstrated With a Novel Prep-Less X-Ray Imaging Capsule for Colon Cancer Screening," Gastroenterology, vol. 146, no. 5, p. S142, 2014. View Article
[3] G. Vanbiervliet, J.-M. Gonzalez and M. Barthet, "Endoscopy Innovations," Gastrointestinal Endoscopy, vol. 80, no. 3, pp. 380-383, 2014. View Article
[4] A. Mercuri, A. Passalacqua, M. S. J. Wickham, R. M. Faulks, D. Q. M. Craig and S. A. Barker, "The effect of composition and gastric conditions on the self-emulsification process of ibuprofen-loaded self-emulsifying drug delivery systems: a microscopic and dynamic gastric model study," Pharmaceutical Research, vol. 28, no. 7, pp. 1540-1551, 2011. View Article
[5] M. Vardakou, A. Mercuri, T. A. Naylor, D. Rizzo, J. M. Butler, P. C. Connolly, M. S. Wickham and R. M. Faulks, "Predicting the human in vivo performance of different oral capsule shell types using a novel in vitro dynamic gastric model," International Journal of Pharmaceutics, vol. 419, no. 1-2, pp. 192-199, 2011. View Article
[6] N. Inou and Y. Umetani, "Small intestinal movements in vivo and the neuro-mechanical control mechanisms," in International Symposium on Autonomous Decentralized Systems. Proceedings ISADS 93., Kawasaki, 1993. View Article
[7] S. Maqbool, H. P. Parkman and F. K. Friedenberg, "Wireless Capsule Motility: Comparison of the SmartPill® GI Monitoring System with Scintigraphy for Measuring Whole Gut Transit," Digestive Diseases and Sciences, vol. 54, no. 10, pp. 2167-2174, 2009. View Article
[8] A. M. Stokes, N. L. Lavie, M. L. Keowen, L. Gaschen, F. P. Gaschen, D. Barthel and F. M. Andrews, "Evaluation of a wireless ambulatory capsule (SmartPill®) to measure gastrointestinal tract pH, luminal pressure and temperature, and transit time in ponies," Equine Veterinary Journal, vol. 44, no. 4, pp. 482-486, 2012. View Article